In this article I explore a topic of which everyone, more or less, have a summary knowledge that comes from the school: the inner ear and in particular the cochlea.
Before going into the details of the structure and function of the cochlea, I would only give some hints on other components of the auditory system. The organ of hearing is in fact a particularly complex system and it would be a shame not to say a few words about its other constituents.
The outer ear, at first sight, is little more than a funnel. The "little more than" actually hides subtleties that would be excessive to study here. Suffice it to say that the particular shape of the ear creates variations in the sound signal that the brain uses to figure out if the sound source is behind, above or in front of our head.
The ear canal itself, or external auditory meatus, at first sight, is little more than a pipe, but in reality it is a tuned duct centered on the frequencies of the auditory spectrum, where lies the human voice.
The middle ear appears already to be a more sophisticated structure. The eardrum and the three ossicles transform the oscillations of the air into mechanical oscillations.
The eardrum directly interfaces with the environment, while the three ossicles perform a real impedance adaptation by taking the relatively large vibration of the tympanic membrane and transform them into oscillations of less amplitude but greater pressure, able to put in motion the cochlear mechanism described later.
But the main topic of this article is the cochlea, which is the organ through which these vibrations are converted into nerve impulses, and that is what we will focus on, starting next paragraph.
The cochlea: physiology
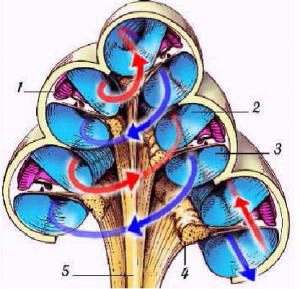
The two membranes then divide the cochlea into three compartments said scales: the scala vestibuli, the scala media and the scala tympani.
The scala vestibuli and tympani are linked together at the end of the cochlea (helicotrema) and contain a fluid called perilymph .
The scala media instead ends in dead-end and contains a fluid called endolymph.
In the pictures below you can see a section of the cochlea imagining that it had been "rolled out". The arrows show the path of the vibrations that propagate along the ducts.
The scala tympani has an opening on one side called oval window where is connected to the stapes (stirrup). This is the entry point of the sound waves that are transmitted by the ossicular chain to the perilymph. The vibrations are transmitted along the canal of the scala vestibuli up to helicotrema and from there they go to the scala tympani where continue backward until another window, that opens to the middle ear, and is called the round window. The round window is closed by a cartilaginous membrane which allows to cushion the vibrations of the perilymph.
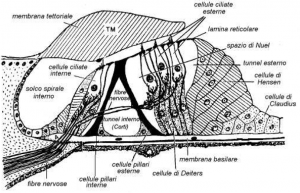
The two types of hair cells have two slightly different functions.
The Outer Hair Cells (OHC) have an active role in the propagation of vibration. In fact, when excited by the vibration of membranes, they contract emitting in turn a vibration proportional to excitation, keeping keeping in vibration the tectorial membrane. This feedback mechanism makes sure that the vibrations, which would normally be damped by the viscosity of the cochlear fluid, are maintained for a longer time by providing a gain of about 100 times (40dB). The mechanical activity of the outer hair cells also produce oscillations that propagate in a retrograde manner until they reache the eardrum. When the eardrum is put into oscillation, are produced the so-called 'otoacoustic emissions', which in recent years have been widely used as a screening method.
The Inner Hair Cells (IHC), represent the real receptor cells, being the only synaptically connected with the fibers of the auditory nerve that carries the acoustic information, transformed into electrical code (action potentials), to the central structures.
What we hear depends on how the mechanical stress of the vibrations affects the Inner Hair Cells. As you can guess from the description of the system, however, the cells do not simply pick up the sound vibrations, that are transmitted from the bracket to the oval window, but they rather react to the vibration of the whole system where they are physically anchored.
So, to understand the functioning of the system, we must first understand what happens when a sound puts in vibration the various membranes of the cochlea.
The cochlea: operating principle
Thanks to what we know of physics and acoustics in general, we know that the sound is constituted by the propagation of an oscillation in an elastic medium such as air or water or even a metal.
Who practice of electronics knows that to this oscillation, by the use of a suitable transducer, a microphone, you can match an analogous electrical oscillation. Who practice of computer science knows that this electrical signal can match a numeric value, and the succession in time of many numerical values is representative of the variation of the original signal. This process can be followed in reverse and end up with the oscillating membrane of another appropriate transducer, the speaker that reproduces the sound to start.
The above is the principle on which they are based all digital audio devices of which today we experience, from CDs to MP3 players.
Having seen how the devices that operate in this manner are common and widespread, we would think that in the cochlea there is not more than the equivalent of an organic microphone that adjusts a nerve signal in proportion to oscillation that is transmitted by the ossicles. Then, as in MP3 players there is a discretization of the signal (that is, a precise numerical value is assigned to the intensity of the signal at a given instant), you may think that the hair cells simply emit discrete discharges in proportion to the intensity of vibration which they are subjected.
This is only partly true. The reality is a bit more complicated. Let's see why.
By convention, we assume that the spectrum of audible by humans extends from 20 to 20,000. Honestly, I doubt that most of the people is really able to hear anything above 16kHz, to be optimistic.
The Shannon's theorem tells us that, in order to reconstruct a wave after it has been sampled, it is necessary that the sampling frequency is at least twice the maximum frequency that you want to achieve. This is the reason why the audio CD are sampled with a frequency of 44.1kHz, to be able to play up to 20kHz. In other words, for every second of music to be stored on an electronic medium, are written 44100 numeric values. In other words, between a value and the next, there is a time interval of about two hundred thousandths of a second. In the images to the right is represented the transition from an analog signal, at the top represented by a continuous curve, as can be the pressure variation of the Air on the airdrum vs. time, and below the same sampled signal. Instead of a continuous curve we have a set of values, equally spaced in time, which represent, in the previous example, the value of the pressure at regular intervals.
Since our nervous system is made up of neurons, our cochlear transducer is in need of actually make a sampling of the signal, because neurons operate in discrete pulses, but collides with the physiological limit of the neurons themselves, which are able to generate a pulse at intervals to a minimum of a few tenths of a millisecond.
This poses a major limitation to the maximum frequency attainable. In fact, the neurons are able to perform a synthesis of the two effects: the tone coding via the tonotopic arrangement along the basement membrane, of which we speak in the next section, and a time coding using a system of phase lock.
Moreover, even if the inner ear was able to really achieve a sampling of the signal at that frequency, this flow of information, once received by the brain, it should be subjected to a processing extremely onerous. In fact, without imagining a re-conversion of the signal into an analogic one, the brain would be to perform some discrete Fourier transforms in order to filter the signal and extract the information, processing tens of thousands of values per second.
With the available hardware, the nature would not have been able to get a working system in real time in the same way they work today electronic devices.
Then th nature followed a different path: she created a mechanical spectrum analyzer, or electromechanical if you prefer.
The mechanism of operation of the cochlea is not that of a microphone which detects the sound intensity moment by moment and associates some kind of nervous stimulation. It is instead a system that associates specific nervous stimuli to each frequency present in the perceived sound!That is, at a given moment, for example, if we are listening to the sound of a contrabass, there will be some hair cells, dedicated to low frequencies, that will send a strong impulse to the brain, plus some impulse, much less intense, produced by the cells dedicated to the high frequencies, which are excited by the sound of the rubbing of the bow's horsehair across the strings.
That above is the operating principle of our biological spectrum analyzer. In the next section we will see how the nature has achieved this goal.
The cochlea: operation in detail
The purpose of having groups of cells excited selectively as a function of the sound frequency is obtained through the physical conformation of the basement membrane.
The basement membrane is a membrane formed from elastic fibers stretched between bone ridges projecting towards the interior of the duct. They are thick and short in the peripheral zone and become longer as the pipe is wound tightly inwards.
In the images are visible a schematization of the longitudinal section of the cochlea, that represents the trend of the fibers, and the width of the membrane itself and a cross section in which is shown schematically the variation of the thickness of the membrane.
As we said before the waves enter the cochlea through the oval window, which supports the stapes. A pressure wave will produce a deflection of the membrane towards the scala tympani, and vice versa. Due to its structure, however, the basilar membrane will not react the same way along all its length to the various stresses. The change of mass and stiffness along the membrane causes at each point the resonance frequency to be different. In particular, the high frequencies will make vibrate more the portion of the membrane closest to the oval window, while the lower frequencies will make vibrate the more distant portion, nearest to the helicotrema.Thanks to this particular structure of the membrane, the elastic stiffness is not constant, but is about 50000 times larger at the base than the apex decreasing with approximately exponential law.
In the following image you can see the pattern of the resonance frequencies as suggested by Hermann von Helmholtz who is the scholar who first proposed this model.
When a complex sound, consisting of different frequencies, enters the cochlea, the result will be that different points of the basilar membrane will vibrate with different amplitudes in function of the spectral composition of the input signal, stressing in a different way the hair cells which are distributed along the membrane. The fact that the sensitive cells are excited by different tones at different points makes that their distribution is called tonotopic. This organization of nerve fibers is preserved all the way to the brain and the primary auditory cortex too retains a tonotopic structure.
Bibliography
Fisica Onde Musica
Università "Federico II" di Napoli
- Otoemissioni Acustiche - A.R. De Caria
Hello @step73! This is a friendly reminder that you have 3000 Partiko Points unclaimed in your Partiko account!
Partiko is a fast and beautiful mobile app for Steem, and it’s the most popular Steem mobile app out there! Download Partiko using the link below and login using SteemConnect to claim your 3000 Partiko points! You can easily convert them into Steem token!
https://partiko.app/referral/partiko
Downvoting a post can decrease pending rewards and make it less visible. Common reasons:
Submit
Congratulations @step73! You received a personal award!
You can view your badges on your Steem Board and compare to others on the Steem Ranking
Vote for @Steemitboard as a witness to get one more award and increased upvotes!
Downvoting a post can decrease pending rewards and make it less visible. Common reasons:
Submit