This article is part of a series about the basics of genetic engineering with CRISPR, if you are not familiar with the subject, I strongly encourage you to read this article first.
We now know how the CRISPR/Cas9 system allows scientists to specifically cut DNA at a desired site inside of virtually any living cell (even though it is often not that easy). While this was possible before with other technologies, CRISPR made the whole process a lot easier and more efficient. This might sound like a limited use for such a hyped technology, but as we will see the ability to specifically induce breaks in a DNA molecule opens the door to many useful applications.
How do cells deal with DNA damages?
Living cells are incredibly sensitive to DNA breaks. The genetic information encoded in our chromosomes contains all the instructions necessary to preform essential cellular functions. If this information is damaged or lost, the cell could simply die or in some cases acquire abnormal traits, which could result in the development of a tumor (which is generally not a good thing). If you combine that with the fact that DNA molecules are constantly subjected to chemical or physical stress, we end up with a serious problem. Indeed, approximately 10 DNA double strand breaks occur in each of our cells every day! This could happen spontaneously during normal cellular processes like cell division or because of external agents such as tobacco smoke, UV light, radiations etc…
Not only that, but double strand breaks are not the only damages that could occur on the DNA molecule! Here are some examples:
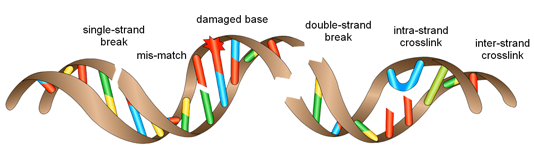
Luckily for us, our cells have evolved to efficiently repair these DNA breaks and different systems exist in mammalian cells to maintain the integrity of the genome. Some of them are active throughout the cell cycle and others only at certain times or in certain tissues. Most of these mechanisms are not fully understood to this day, and multiple pathways generally act in parallel inside a single cell so I am not going to list the specificities of each repair pathway. However, it is important to know that in general a break could be either repaired precisely without any modification to the nucleotide sequence or on the contrary by introducing some small modifications to the sequence (some letters are added, changed or lost in the process). These mutations are generally harmless when they happen in regions of the genome that do not contain genes (which corresponds to approximately 98% of the total size of the genome!*). This explains why everyone does not develop a new cancer or genetic disease every day.
Knock-in/Knock-out
However, these mutations could also be exploited to disrupt a gene: by simply cutting DNA in the coding region of a gene, the small mutations induced by the repair machinery of the cell could make the information carried by the gene completely unreadable, thus simulating the absence of this gene. This application is called gene knock-out (KO) and is very useful to study the role of a gene: by knocking-out an unknown gene and observing the effect on the cell or on the whole organism (if the modification was performed at the scale of a tissue or at an early embryonic stage), it is possible to determine the molecular function of this specific gene.
Another common application made simpler by CRISPR is on the contrary the introduction of an exogenous sequence in a genome, like for example a gene from another species or a healthy allele to replace a mutation associated with a disease. This process called gene (or sequence) knock-in (KI) requires to provide the cell with a DNA molecule (which could be under different forms) containing similarities with the target sequence as well as the desired modification. The repair machinery of the cell will thus recognize this foreign molecule as homologous to the damaged region and will use it as a template to precisely repair the break through a mechanism known as Homology Directed Repair (HDR).
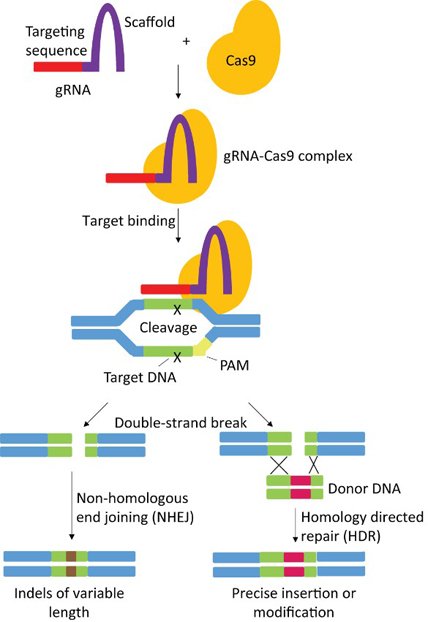
We now know how CRISPR made it easier, faster and cheaper to insert or delete a specific sequence in the genome of a cell. This could be virtually done in any organism; however the DNA repair mechanisms sometimes differ greatly between different organisms, which could be a limitation (bacteria for example are mostly unable to perform Homology Directed Repair).
Some limitations
While it is now clear how CRISPR is an incredibly valuable tool for academic research and the industry (to insert a gene in a plant to make it resistant to drought for example), things are not that simple in the world of biology. As mentioned earlier, the mechanisms used by cells to repair DNA are poorly understood and often variable depending on the considered cell and experimental conditions.
This could, in the best case scenario, make the modification process somewhat inefficient or at worst completely unpredictable. Furthermore, introducing the CRISPR/Cas9 complex into the cells is not always easy. Most cells do not like unrequested foreign molecules penetrating their membrane, and this could result in an important toxicity for the cells.
Finally, the recognition of its target by the CRISPR system is accurate but not perfect. Genomes of higher organisms are indeed very large and contain intricate networks of repeated sequences, which could lead to the cutting of DNA at “off-target” sites that are similar or simply identical to the desired target region. We will discuss these limitations and what they mean for the applications of CRISPR in medicine, pharmacology, biological research, agro-engineering and others in the next article.
(*) The fraction of the genome occupied by genes and what could be considered as a “coding” regions is still subject to controversy. I voluntarily simplified and considered only protein-coding regions here. This does not mean that 98% of the genome is useless (or composed of junk DNA as thought before)!
Sources and further reading
- A review article giving a good overview of DNA repair mechanisms: http://www.nature.com/doifinder/10.1038/ncb3452
- Articles taking a deeper look at DNA repair pathway choice at DNA double strand breaks: http://www.annualreviews.org/doi/abs/10.1146/annurev-genet-110410-132435 https://www.sciencedirect.com/science/article/pii/S0968000415001589
- An article showing interesting characteristics of the repair outcomes observed at the Cas9 cut site: http://linkinghub.elsevier.com/retrieve/pii/S1097276516303252
Good job buddy!
Downvoting a post can decrease pending rewards and make it less visible. Common reasons:
Submit
Thanks Francesco :D
Downvoting a post can decrease pending rewards and make it less visible. Common reasons:
Submit