Invest in renewable energy? The answer may be nuclear physics
They have passed 13,700 million years since the 'big bang', the ephemeral instant in which, with a big bang, they began to originate the chemical elements that make up the universe. Accurately understanding the origin of matter is one of the most arduous tasks physicists face today.
Nuclear Physics
Nuclear Physics studies the structure of atomic nuclei, which contain practically all of the mass of matter and where reactions that make stars shine or produce energy occur. The protons and neutrons that make up the nucleus of the atom are linked by the short-range nuclear interaction. The balance between repulsion between protons and the nuclear attraction of protons and neutrons gives rise to all known nuclei.
To know how the different elements appear, we must know how all the nuclei were created from primordial hydrogen. At the origin of the Universe only hydrogen and some light nuclei such as helium were created. The largest nuclei were created inside the stars or in violent phenomena such as supernova explosions. The creation of cores heavier than iron is more complex, since these nuclei can not be created by the mere fusion of the nuclear fuel of the stars.
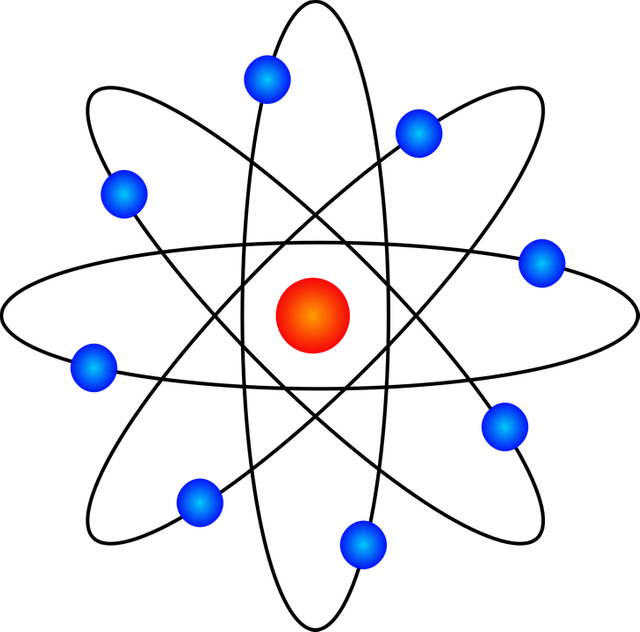
The history of nuclear physics
The history of nuclear physics as a discipline distinct from atomic physics begins with the discovery of radioactivity by Henri Becquerel in 1896, while investigating the phosphorescence of uranium in salts. The discovery of the electron by JJ Thomson, a year later, was an indication that the atom had an internal structure.
At the turn of the 20th century the accepted model of the atom was JJ Thomson's "plum pudding" model in which the atom was a large ball of positive charge with the small negatively charged electrons embedded within it. With the turn of the century, physicists had also discovered three types of radiation from atoms, which they called alpha, beta and gamma radiation.
Experiments in 1911 by Lise Meitner and Otto Hahn, and by James Chadwick in 1914 discovered that the beta decay of the spectrum was continuous and not discrete. That is, the electrons are expelled from the atom with a range of energies, instead of the discrete amounts of energy that were observed in gamma and alpha decays. This was a problem for nuclear physics at the time, since it indicated that energy is not conserved in these decays.
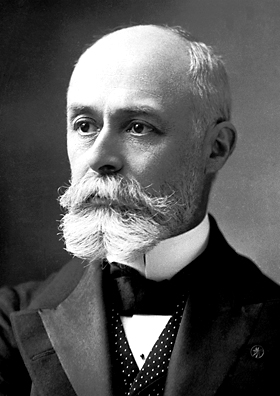
The stability of the nucleus can not be explained by its electrical action. Moreover, the repulsion between the protons would produce its disintegration. The fact that there are protons and neutrons in the nucleus is an indicator that there must be another interaction stronger than the electromagnetic one that is not directly related to electrical charges and that is much more intense. This interaction is called nuclear and is the one that predominates in the nucleus.
Nuclear energy
Nuclear energy can be used to produce electricity. But first the energy must be released. This energy can be obtained in two ways: nuclear fusion and nuclear fission. In nuclear fusion, the energy is released when the nuclei of the atoms combine or fuse together to form a larger nucleus. This is how the sun produces energy. In nuclear fission, the nuclei separate to form smaller nuclei, releasing energy. Nuclear power plants use nuclear fission to produce electricity.
When one of these two nuclear reactions occurs (nuclear fission or nuclear fusion) the atoms experience a slight loss of mass. This mass that is lost becomes a large amount of heat energy and radiation, as Albert Einstein discovered with his famous equation E = mc². The heat energy produced is used to produce steam and generate electricity. Although the production of electrical energy is the most common utility that is given to nuclear energy, it can also be applied in many other sectors, such as medical or environmental applications.
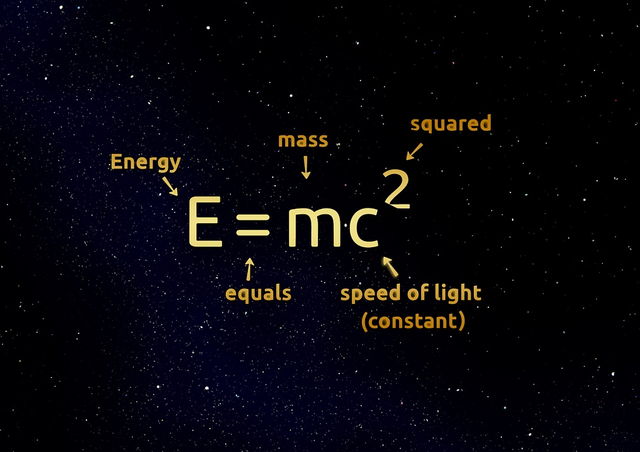
Modern nuclear physics
A heavy nucleus can contain hundreds of nucleons that mean that with a certain approximation it can be treated as a classical system, instead of a quantum mechanics. In the result of placing the liquid model, the nucleus has an energy that arises partly from the surface tension and in part from the electrical repulsion of the protons. The liquid release model is capable of reproducing many characteristics of the nuclei, including the general trend of binding energy with respect to mass number, as well as the phenomenon of nuclear fission.
To study these processes and determine why some elements are more abundant than others, extreme temperature and pressure conditions are recreated in the laboratory that reproduce the reactions of creating heavy nuclei. These experiments are carried out at facilities such as ISOLDE at CERN, or FAIR, which is being built at the GSI laboratory in Germany.
Nuclear Physics not only allows us to answer questions about how matter is formed or how the Universe has evolved. It has also allowed the development of applications ranging from therapies and medical diagnosis through techniques such as positron emission tomography (PET) or hadrontherapy, to the generation of energy or the analysis of works of art. Currently, more than half of the particle accelerators scattered around the world are in hospitals for use in medicine.
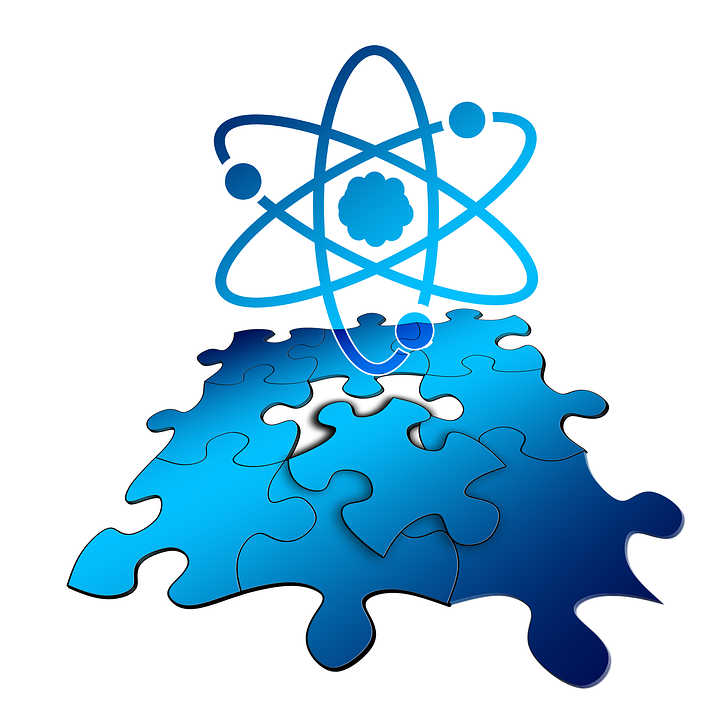