Welcome to the knowledge of science, today in this interesting publication, I will talk about DNA, this acid that is so important for our body as it is considered the engine of our entire body, now many times we ask what DNA is, or how this is structured, here in this article you can clarify all your questions, so I invite you to see this interesting publication ..
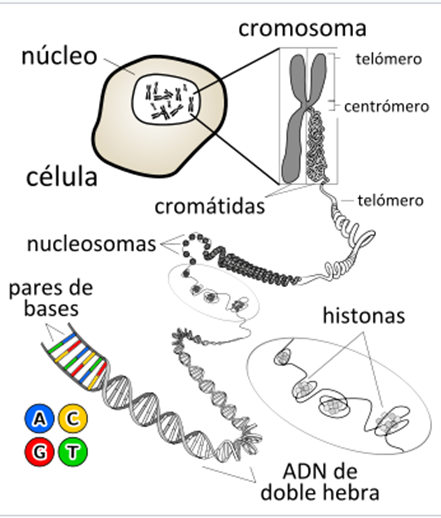
Deoxyribonucleic acid
Deoxyribonucleic acid, abbreviated as DNA, is a nucleic acid that contains the genetic instructions used in the development, functioning of all living organisms and some viruses, it is also responsible for its hereditary transmission. The main function of the DNA molecule is the long-term storage of information to build other components of cells, such as proteins and RNA molecules. The DNA segments that carry this genetic information are called genes, but the other DNA sequences have structural purposes or take part in the regulation of the use of this genetic information.
From the chemical point of view, DNA is a polymer of nucleotides, that is, a polynucleotide. A polymer is a compound formed by many simple units connected to each other, as if it were a long train formed by wagons. In DNA, each wagon is a nucleotide, and each nucleotide, in turn, consists of a sugar (deoxyribose), a nitrogenous base (which can be adenine → A, thymine → T, cytosine → C or guanine → G ) and a phosphate group that acts as a hook for each car with the next. What distinguishes a wagon (nucleotide) from another is, then, the nitrogenous base, and therefore the DNA sequence is specified by naming only the sequence of its bases. The sequential arrangement of these four bases along the chain (the ordering of the four types of wagons along the entire train) is what encodes the genetic information: for example, a DNA sequence can be ATGCTAGATCGC .. In living organisms, DNA is presented as a double chain of nucleotides, in which the two strands are linked together by connections called hydrogen bonds.
History of genetics
DNA isolated him for the first time, during the winter of 1869, the Swiss physician Friedrich Miescher while working at the University of Tübingen. Miescher performed experiments on the chemical composition of pus from discarded surgical bandages when he noticed a precipitate of an unknown substance that he chemically characterized later, called it nuclein, because it had been extracted from cell nuclei. years of research to identify the components and structure of nucleic acids.
The biological function of DNA began to be elucidated in 1928, with a basic series of experiments in modern genetics carried out by Frederick Griffith, who was working with "smooth" (S) or "rough" (R) strains of the Pneumococcus bacteria (causative of pneumonia), according to the presence (S) or not (R) of a sugar capsule, which is the one that confers virulence (see also Griffith experiment). The injection of live S pneumococci into mice killed them, and Griffith observed that if mice were injected with live R pneumococci or with heat killed S pneumococci, the mice did not die. However, if you injected both live R pneumococci and dead S pneumococci, the mice died, and live S pneumococci could be isolated in your blood. Since the dead bacteria could not have multiplied within the mouse, Griffith reasoned that some kind of change or transformation from one bacterial type to another must occur through a transfer of some active substance, which he called the transforming principle.
Physical and chemical properties
DNA is a long polymer formed by repetitive units, the nucleotides.A double strand of DNA measures from 22 to 26 angstroms (2.2 to 2.6 nanometers) wide, and one unit (one nucleotide) measures 3.3 Å (0.33 nm) long. Although each individual repeating unit is very small, the DNA polymers can be huge molecules that contain millions of nucleotides. For example, the longest human chromosome, chromosome number 1, has approximately 220 million base pairs.
In living organisms, DNA does not usually exist as an individual molecule, but rather as a pair of closely associated molecules. The two strands of DNA are curled on themselves forming a kind of spiral staircase, called double helix. The double helix structure model was proposed in 1953 by James Watson and Francis Crick (the article "Molecular Structure of Nucleic Acids: A Structure for Deoxyribose Nucleic Acid" was published on April 25, 1953 in Nature), after obtaining a image of the double helix structure thanks to the X-ray refraction done by Rosalind Franklin The success of this model was in its consistency with the physical and chemical properties of DNA. The study also showed that base complementarity could be relevant in its replication, and also the importance of the base sequence as a carrier of genetic information.Each unit that repeats, the nucleotide, contains a segment of the structure of support (sugar + phosphate), which holds the chain together, and a base, which interacts with the other strand of DNA in the helix. In general, a base linked to a sugar is called a nucleoside and a base linked to a sugar and to one or more phosphate groups is called a nucleotide.
Components
Support structure: The support structure of a DNA strand is formed by alternating units of phosphate and sugar (deoxyribose) groups. The sugar in DNA is a pentose, specifically, deoxyribose.
- Phosphoric acid:
Its chemical formula is H3PO4. Each nucleotide can contain one (monophosphate: AMP), two (diphosphate: ADP) or three (triphosphate: ATP) phosphoric acid groups, although as nucleic acid constituent monomers only appear in the form of nucleoside monophosphate.
- Deoxyribose:
It is a monosaccharide of 5 carbon atoms (a pentose) derived from ribose, which is part of the nucleotide structure of DNA. Its formula is C5H10O4. One of the main differences between DNA and RNA is sugar, because in RNA the 2-deoxyribose in DNA is replaced by a pentose alternative, ribose.
The sugar molecules are bound together through phosphate groups, which form phosphodiester bonds between the third carbon atoms (3 ',' three cousins ') and fifth (5', 'five cousins') of two adjacent sugar rings . The formation of asymmetric links implies that each strand of DNA has a direction. In a double helix, the direction of the nucleotides in one strand (3 '→ 5') is opposite to the direction in the other strand (5 '→ 3'). This organization of the DNA strands is called antiparallel; they are parallel chains, but with opposite directions. In the same way, the asymmetric ends of the DNA strands are called the 5 'end ("five prime") and the 3' end ("three prime"), respectively.
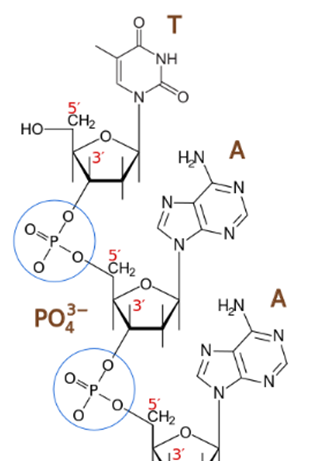
Phosphodiester linkage. The phosphate group (PO43-) joins the 5 'carbon of the sugar of one nucleoside with the carbon 3' of the next.Image source: https://es.wikipedia.org
Nitrogenous bases:
The four major nitrogenous bases found in DNA are adenine (A), cytosine (C), guanine (G) and thymine (T). Each of these four bases is linked to the sugar-phosphate framework through the sugar to form the complete nucleotide (base-sugar-phosphate). The bases are heterocyclic and aromatic compounds with two or more nitrogen atoms, and, within the majority bases, are classified into two groups: the purine bases or purines (adenine and guanine), derived from the purine and formed by two joined rings among themselves, and pyrimidine bases or pyrimidine bases or pyrimidines (cytosine and thymine), derived from pyrimidine and with a single ring.25 In nucleic acids there is a fifth pyrimidine base, called uracil (U), which normally occupies the RNA differs from thymine in that it lacks a methyl group in its ring. Uracil is not usually found in DNA, it only appears rarely as a residual product of the degradation of cytosine by oxidative deamination processes.
Timina:
In the genetic code it is represented by the letter T. It is a pyrimidine derivative with an oxo group in positions 2 and 4, and a methyl group in position 5. It forms the nucleoside thymidine (always deoxythymidine, since it only appears in DNA ) and the nucleotide thymidylate or thymidine monophosphate (dTMP). In DNA, thymine is always paired with the adenine of the complementary chain by means of 2 hydrogen bonds, T = A. Its chemical formula is C5H6N2O2 and its nomenclature 2,4-dioxo, 5-methylpyrimidine.
Cytosine:
In the genetic code it is represented by the letter C. It is a pyrimidine derivative, with an amino group in position 4 and an oxo group in position. It forms the nucleoside cytidine (deoxycytidine in DNA) and the nucleotide cytidylate or (deoxy) cytidine monophosphate (dCMP in DNA, CMP in RNA). The cytosine is always paired in the DNA with the guanine of the complementary chain by a triple bond, C≡G. Its chemical formula is C4H5N3O and its nomenclature 2-oxo, 4 aminopirimidina. Its molecular mass is 111.10 units of atomic mass. Cytosine was discovered in 1894, by isolating it from the tissue of the sheep thymus.
Adenine:
In the genetic code it is represented by the letter A. It is a derivative of purine with an amino group in position 6. It forms the nucleoside adenosine (deoxyadenosine in DNA) and nucleotide adenylate or (deoxy) adenosine monophosphate (dAMP, AMP ). In the DNA it is always paired with the thymine of the complementary chain by means of 2 hydrogen bonds, A = T. Its chemical formula is C5H5N5 and its nomenclature 6-aminopurine. Adenine, together with thymine, was discovered in 1885 by the German physician Albrecht Kossel.
Guanina:
In the genetic code it is represented by the letter G. It is a purine derivative with an oxo group in position 6 and an amino group in position 2. It forms the nucleoside (deoxy) guanosine and the nucleotide guanylate or (deoxy) guanosine monophosphate ( dGMP, GMP). Guanine is always paired in the DNA with the cytosine of the complementary strand by three hydrogen bonds, G≡C. Its chemical formula is C5H5N5O and its nomenclature 6-oxo, 2-aminopurine.
There are also other nitrogenous bases (so-called minor nitrogen bases), derived naturally or synthetically from some other major base. For example, hypoxanthine, relatively abundant in tRNA, or caffeine, both derived from adenine; others, such as acyclovir, derived from guanine, are synthetic analogs used in antiviral therapy; others, like one of the derivatives of uracil, are antitumor.
Structure
DNA is a double-stranded molecule, that is, it is formed by two chains arranged antiparallel and with the nitrogenous bases facing each other. In its three-dimensional structure, different levels are distinguished:
Primary structure
Sequence of chained nucleotides. It is in these chains where the genetic information is found, and since the skeleton is the same for everyone, the difference in information lies in the different sequence of nitrogenous bases. This sequence presents a code, which determines one information or another, according to the order of the bases.
Secondary structure
It is a double helix structure. It allows explaining the storage of genetic information and the mechanism of duplication of DNA. It was postulated by Watson and Crick, based on the X-ray diffraction that Franklin and Wilkins had made, and Chargaff's base equivalence, according to which the sum of adenines plus guanines equals the sum of thymines plus cytosines.
It is a double chain, dextrorotatory or levorotatory, depending on the type of DNA. Both chains are complementary, since the adenine and the guanine of a chain are united, respectively, to the thymine and the cytosine of the other. Both chains are antiparallel, since the 3 'end of one faces the 5' end of the homologue.
There are three DNA models. Type B DNA is the most abundant and is the one with the structure described by Watson and Crick.
Tertiary structure
It refers to how DNA is stored in a small space, to form chromosomes. It varies according to whether it is prokaryotic or eukaryotic organisms:
In prokaryotes, DNA folds like a super-helix, generally in a circular form and associated with a small amount of proteins. The same occurs in cellular organelles such as mitochondria and chloroplasts.
In eukaryotes, since the amount of DNA of each chromosome is very large, the packing must be more complex and compact; for this, the presence of proteins, such as histones and other proteins of a non-histological nature, is needed (in spermatozoa these proteins are protamines).
Quaternary structure
The chromatin present in the core has a thickness of 300 Å, since the chromatin fiber of 100 Å is wound forming a chromatin fiber of 300 Å. The winding of the nucleosomes is called the solenoid. Said solenoids coil forming the chromatin of the interphase nucleus of the eukaryotic cell. When the cell enters division, the DNA becomes more compact, thus forming the chromosomes.
Double helix structures
DNA exists in many conformations, however, in living organisms only conformations DNA-A, DNA-B and DNA-Z have been observed. The conformation adopted by the DNA depends on its sequence, the amount and direction of supercoiling it presents, the presence of chemical modifications in the bases and the conditions of the solution, such as the concentration of metal ions and polyamines. Of the three conformations , the "B" form is the most common in the conditions existing in the cells, the two alternative double helices of the DNA differ in their geometry and dimensions.
Form "A" is a spiral that turns to the right, wider than "B", with a smaller superficial and wider groove, and a larger, narrower and deeper groove. The "A" form occurs in non-physiological conditions in dehydrated DNA forms, while in the cell it can occur in hybrid DNA-RNA hybrid pairings, as well as in enzyme-DNA complexes.
The DNA segments in which the bases have been modified by methylation can undergo major conformational changes and adopt the "Z" form. In this case, the threads rotate around the axis of the helix in a spiral that turns to the left, the opposite of the more frequent "B" shape. These rare structures can be recognized by specific proteins that bind Z-DNA and are possibly involved in the regulation of transcription.
Quadruplex structures
At the ends of the linear chromosomes there are specialized regions of DNA called telomeres. The main function of these regions is to allow the cell to replicate the chromosomal ends using the telomerase enzyme, since the enzymes that replicate the rest of the DNA can not copy the 3 'ends of the chromosomes.These specialized chromosomal terminations also protect the ends of DNA, and prevent DNA repair systems in the cell from processing them as damaged DNA that must be corrected.In human cells, telomeres are long stretches of single-stranded DNA that contain a few thousand repeats of a single TTAGGG sequence.
These guanine-rich sequences can stabilize the chromosomal ends by forming stacked game structures of four-base units, rather than the base pairs normally found in other DNA structures. In this case, four guanine bases form units with a flat surface that are stacked one on top of the other, to form a stable quad-G structure. These structures are stabilized by forming hydrogen bonds between the ends of the bases and the chelation of an ionic metal in the center of each four-base unit, other structures can also be formed, with the four-base central set coming from, or of a single strand folded around the bases, or of several different parallel strands, so that each contributes a base to the central structure.
Applications
genetic engineering
Research on DNA has a significant impact, especially in the field of medicine, but also in agriculture and livestock (where the objectives are the same as with traditional techniques that man has been using for millennia - domestication, selection and directed crossings - to obtain more productive varieties of animals and plants). Modern biology and biochemistry make intensive use of recombinant DNA technology, introducing genes of interest in organisms, with the aim of expressing a specific recombinant protein, which can be:
isolated for later use: for example, microorganisms can be transformed into authentic factories that produce large quantities of useful substances, such as insulin or vaccines, which are subsequently isolated and used therapeutically.
necessary to replace the expression of a damaged endogenous gene that has given rise to a pathology, which would allow the reestablishment of the activity of the lost protein and eventually the recovery of the normal, non-pathological physiological state. This is the goal of gene therapy, one of the fields in which we are actively working in medicine, analyzing advantages and disadvantages of different gene administration systems (viral and non-viral) and the mechanisms for selecting the integration point of the gene. the genetic elements (different for viruses and transposons) in the target genome. In this case, before considering the possibility of performing gene therapy in a certain pathology, it is essential to understand the impact of the gene of interest in the development of this pathology, for which it is necessary to develop an animal model, eliminating or modifying said gene in a laboratory animal, using the knockout technique. Only in the case that the results in the animal model are satisfactory would proceed to analyze the possibility of restoring the damaged gene by gene therapy.
used to enrich a food: for example, the composition of milk (an important source of protein for human and animal consumption) can be modified by transgenesis, adding exogenous genes and deactivating endogenous genes to improve its nutritional value, reduce infections in the glands mammals, provide consumers with antipathogenic proteins and prepare recombinant proteins for pharmaceutical use.
useful to improve the resistance of the transformed organism: for example in plants you can introduce genes that confer resistance to pathogens (viruses, insects, fungi ...), as well as abiotic stressors (salinity, dryness, heavy metals ...).
References :
Sergio Ferrer. "The true meaning of life." Journal of Feelsynapsis (JoF). : 119-127.
Dahm, R. (2005). «Friedrich Miescher and the discovery of DNA». Dev Biol 278 (2): 274-88. PMID 15680349. doi: 10.1016 / j.ydbio.2004.11.028.
http://www.terradaily.com/reports/Building_Life_On_Earth_999.html DNA discovery data on terradaily.com
Dahm, R. (2008). «Discovering DNA: Friedrich Miescher and the early years of nucleic acid research». Hum Genet 122 (2): 565-581. PMID 17901982.Levene, P. (1919). «The structure of yeast nucleic acid». J Biol Chem 40 (2): 415-24.
Dhanda, J. S .; Shyam, S. Chauhan (22-Feb-2008). «Structural Levels of Nucleic Acids and Sequencing.». In All India Institute of Medical Sciences. Molecular Biology.
(Department of Biochemistry edition). New Delhi - 110 029. (Revised October 7, 2008).
Astbury, W. (1947). «Nucleic acid». Symp. SOC. Exp. Bbl 1 (66).Lorenz, M. G., Wackernagel, W. (1994). «Bacterial gene transfer by natural genetic transformation in the environment». Microbiol. Rev. 58 (3): 563-602. PMID